Zostera marina and anthropogenic nutrient loading
- Nov 30, 2009 - very nice, expand on what any long term implications might be
Z. marina overview:
Zostera marina (Fig. 1) is a marine angiosperm, commonly referred to as eelgrass, that grows in shallow, temperate nearshore (+1.8 to -8.8M in Puget Sound (Mumford 2007)) regions of the Northern Hemisphere (Fig. 2). It is the predominant seagrass species found in Puget Sound (Mumford 2007). It is capable of reproducing both vegetatively by creeping rhizomes and sexually by seed propagation to form carpet-like patches and “meadows” (Touchette 2007). Conservation of
Z. marina is the subject of great concern in the Pacific Northwest because it’s meadow-forming properties “engineer” habitat for a diverse range of marine organisms by providing three-dimensional structure and shelter from predators, stabilizing sediment (contributing to greater water clarity), and attenuating wave energy and currents (Koch 2001). Of particular interest are commercially valuable species like juvenile salmon and rockfish, Dungeness crab, bivalves and other invertebrates, and forage fish that depend on this habitat to thrive (Mumford 2007).
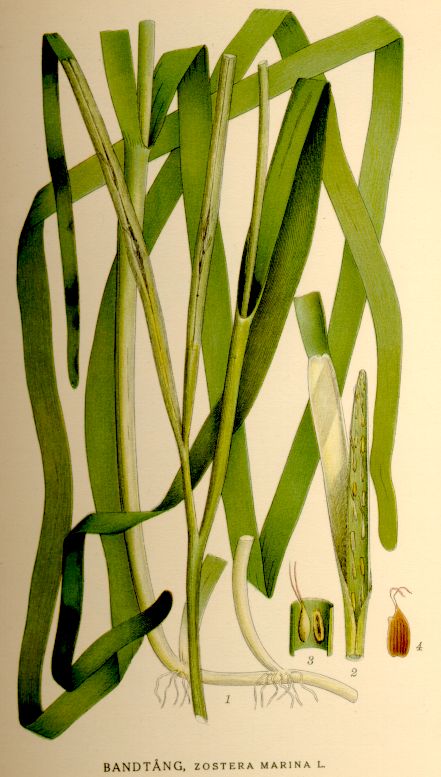 |
Figure 1. Zostera marina (from http://runeberg.org/nordflor/491.html) |
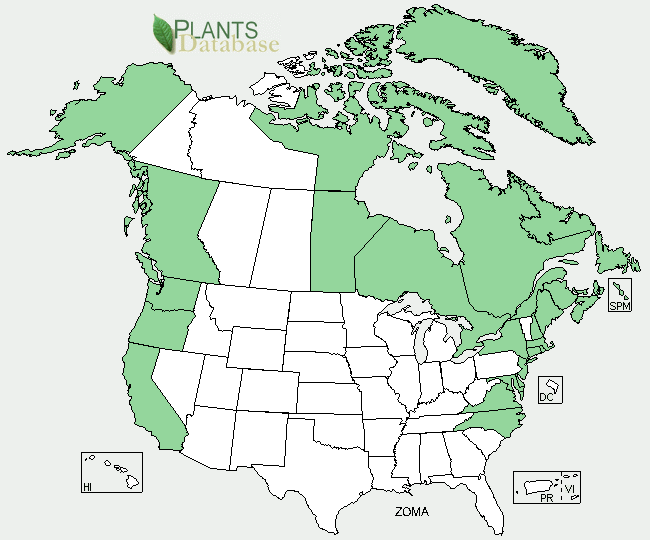 |
Figure 2. Z. marina distribution in North America (http://plants.usda.gov/java/profile?symbol=ZOMA) |
Stressor - anthropogenic nutrient loading:
As a nearshore-dependent species, eelgrasses are also therefore in close proximity to human development, and the myriad changes we impose upon the environment. While a variety of anthropogenic stressors are likely affecting eelgrass populations in Puget Sound and elsewhere, an emerging concern is nutrient loading from anthropogenic sources (Mumford 2007, Burkholder et al. 2007). Non-point source runoff from agricultural use of fertilizers, sewer outfall and faulty septic systems, aquaculture operations, and conversion of evergreen forests to early successional nitrogen-fixing alder forests are all thought to contribute to increases in the amount of nitrogen and phosphorous available in the marine environment. In the absence of human influence, nutrients in the Puget Sound are thought to be available on a cyclic, seasonal basis when upwelling currents bring nutrient-rich byproducts of detrital food webs up from deep bottom waters, and thus, marine plants and algae that are dependent on these nutrients for growth have evolved to persist in low nutrient conditions and make best use of them when available. When nutrients (in this case the focus will be on nitrogen) become available on a continuous basis, and in areas where they may have been absent before, physiological, organismal, and ecological changes are likely to occur.
Physiological Responses:
Seagrasses acquire nitrogen from both the water column (mostly as nitrate, NO3-) through the leaves and from sediment pore water (particularly ammonium, NH4+ in hypoxic or anoxic sediments) through the roots (Touchette 2000). Nitrate and ammonium uptake and assimilation are mediated by the nitrate reductase and glutamine synthetase/glutamate sythase (GS/GOGAT) pathways, respectively (Fig. 3). Evidence suggests that while rates of root uptake of ammonium are reduced when water column Ni is increased, uptake rates in leaves are not changed when pore water Ni is increased to levels that should meet the organism’s requirements (Thursby & Harlin 1982). Additionally, whereas other seagrasses only reduce nitrate during the day, Z. marina reduces nitrate during both day and night if it becomes available (Touchette & Burkholder 2001), and at least under some circumstances appears to have little to no product feedback control limiting the nitrate reductase pathway (Roth & Pregnall 1988). These functions are thought to be adaptations to oligotrophic environments that are N limiting, thus allowing Z. marina leaves to uptake and store even small water column pulses of N at high affinity independent of the sediment nitrogen content (Short & McRoy 1984). These adaptations may become less advantageous as conditions become eutrophic and N is no longer limiting, however. While the most obvious cause of eelgrass decline under eutrophic conditions may be overgrowth, shading, and water quality degradation by dense algal blooms and epiphytes, it has been shown that direct physiological impacts of excess nitrogen uptake can also contribute to poor health and death.
Nitrogen uptake and assimilation are very energetically expensive processes, and pulses of water column nitrogen have been shown to reduce soluable carbon species, increase leaf respiration, and induce internal carbon imbalances and structural weakness in meristematic regions in Z. marina, presumably because carbon and energy are diverted to amino acid synthesis to avoid toxic accumulation of ammonia in the tissues (Touchette et al. 2003, Turpin et al. 1991). This imbalance could result in organism death or growth and reproduction restriction.
While ammonium uptake and assimilation from sediment pore water appear to be under greater product feedback inhibition than nitrate uptake in eelgrass (Touchette 2000), at least one study has indicated that increased sediment organic content can contribute to increased leaf growth relative to root density, resulting at least in some cases in a mismatch between surrounding wave and current energy and the ability of eelgrass shoots to remain attached to the substrate (Wicks et al. 2009).
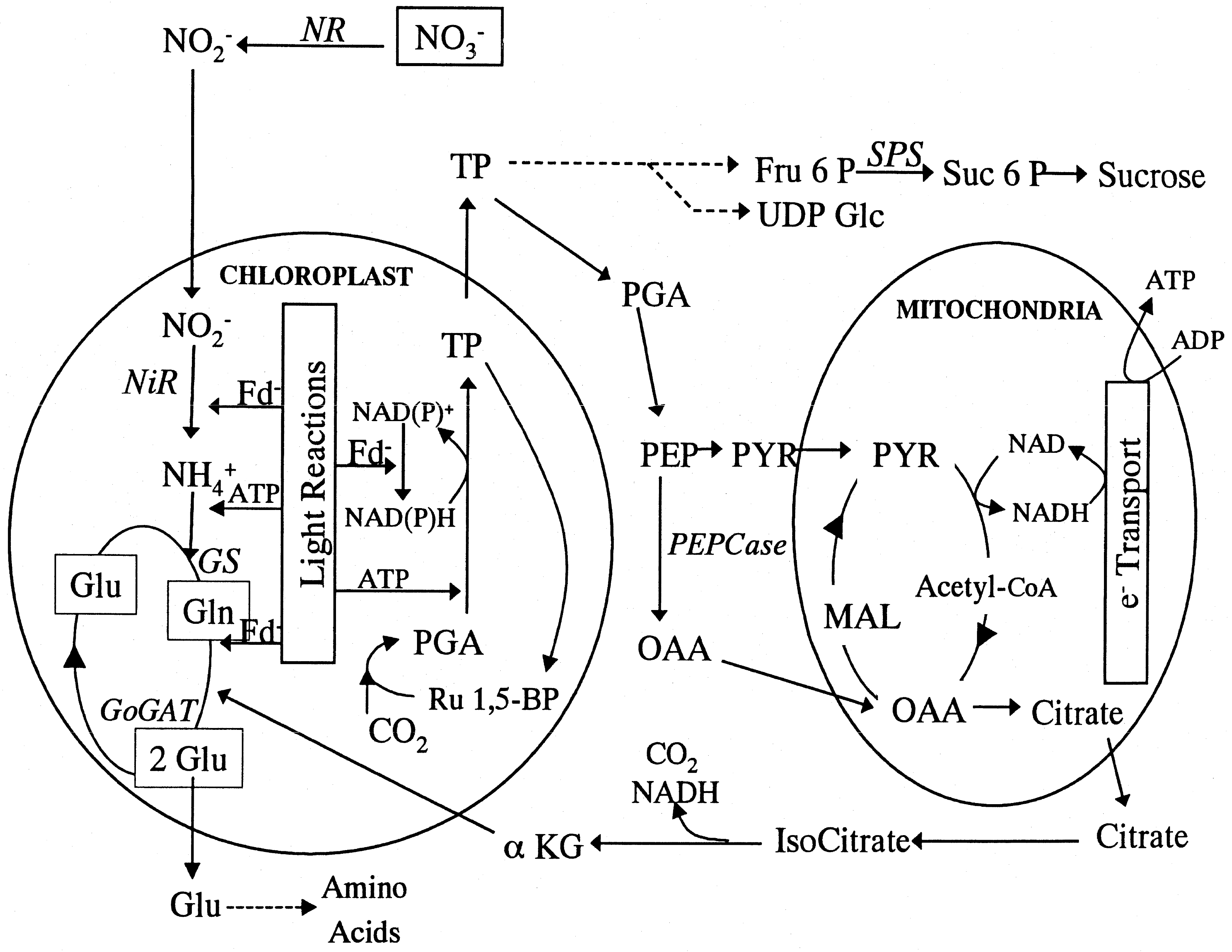 |
Figure 3. Reactions involved in nitrate uptake and assimilation in plant cells. Uptake and assimilation of nitrate as well as ammonium occur primarily in the cytosol and plastids (chloroplasts, mitochondria). As nitrate enters the cell, it can be stored in a vacuole (not shown) or reduced in the cytosol by the enzyme nitrate reductase (NR). The product, nitrite, enters the plastids (usually the chloroplasts) where it is further reduced to ammonium by the enzyme nitrite reductase (NiR). Ammonium then enters the GS/GOGAT cycle, where glutamate is aminated by the enzyme glutamine synthetase (GS) to synthesize glutamine. The addition of carbon skeletons (a-ketoglutarate) allows transamination by glutamate synthase (GOGAT) to produce two glutamate molecules. One of these can be recycled through the GS/GOGAT pathway, whereas the other can be used to form more complex amino acids (adapted from Ferrario-Mery et al., 1997). (from Touchette et al. 2000) |
Potential Implications:
Increasing anthropogenic input of nutrients into waterways and coastal regions is likely to affect marine ecosystems at multiple levels of biological organization. In the case of sumberged aquatic vegetation like seagrasses, modest increases in water column nutrient content may have a beneficial effect by eliminating any growth-rate limitations imposed by restricted N or other nutrients. This fertilization effect will also benefit epiphytic and suspended algal populations, however, which can grow rapidly to large biomass, potentially shading eelgrass to the point of elimination. At higher N concentrations, seagrasses that evolved in nutrient-limited environments and lack feedback control of N uptake may become energetically overburdened by maintaining uptake machinery and processes, and need to divert carbon resources from processes like growth, structural support, and reproduction in order to neutralize toxic products of nitrogen reduction, resulting in poor survival and propagation. It remains to be seen whether sufficient genetic diversity exists in the
Z. marina population to adapt to increased nutrient availability, but given its wide geographic range, it seems likely that organisms from at least a few regions will be able to persist.
Burkholder, J M; Tomasko, D A; Touchette, B W. 2007. Seagrasses and eutrophication. Journal of Experimental Marine Biology and Ecology. 350: 46-72.
Ferrario-Mery, S., Murchie, E., Hirel, B., Galtier, N., Quick, W.P., Foyer, C.H., 1997. Manipulation of the pathways of sucrose biosynthesis and nitrogen assimilation in transformed plants to improved photosynthesis and productivity. In: Foyer, C.H., Quick, W.P. (Eds.), A Molecular Approach To Primary Metabolism in Higher Plants. Taylor & Francis, London, pp. 125–153.
Koch, E W. 2001. Beyond light: physical, geological, and geochemical parameters as possible submerged aquatic vegetation habitat requirements. Estuaries 24, no. 1: 1-17
Mumford Jr., T F. 2007. Kelp and eelgrass in Puget Sound. Technical Report, Puget Sound Nearshore Partnership Report No. 2007-05, Seattle, Washington: Seattle District, U.S. Army Corps of Engineers.
Perez, M; Garcia, T; Invers, O; Ruiz, J M. 2008. Physiological response of the seagrass Posidonia oceanica as indicators of fish farm impact. Marine Pollution Bulletin. 56: 869-879.
Short, F T & McRoy, C P. 1984. Nitrogen uptake by leaves and roots of the seagrass Zostera marina L. Botanica Marina. 17:547-555.
Touchette, B W & Burkholder, J M. 2000. Review of nitrogen and phosphorous metabolism in seagrasses. Journal of Experimental Marine Biology and Ecology. 250: 133-267.
Touchette, B W & Burkholder, J M. 2001. Nitrate reductase activity in a submersed marine angiosperm: controlling influences of environmental and physiological factors. Plant Physiology & Biochemistry, 39:583-593.
Touchette, B W; Burkholder, J M; Glasgow, H B. 2003. Variations in eelgrass (Zostera marina L.) morphology and internal nutrient composition as influenced by increased temperature and water column nitrate. Estuaries. 26:142-155.
Touchette, B W. 2007. Preface: The biology and ecology of seagrasses (special issue). Journal of Experimental Marine Biology and Ecology. 350: 1-2.
Turpin, D H; Vanlerberghe, G C; Amory, A M; Guy, R D. 1991. The inorganic carbon requirements for nitrogen assimilation. Canadian Journal of Botany. 69-1139-1145.
Wicks, C E; Koch, E W; O’Neil, J M; Elliston, K. 2009. Effects of sediment organic content and hydrodynamic conditions on the growth and distribution of Zostera marina. Marine Ecology Progress Series, 378:71-80.
This page was developed as part of the course at the University of Washington: Integrative Environmental Physiology